Scientists Have Found Some of the Genes That Make Us So Complex
The root difference between us and worms.
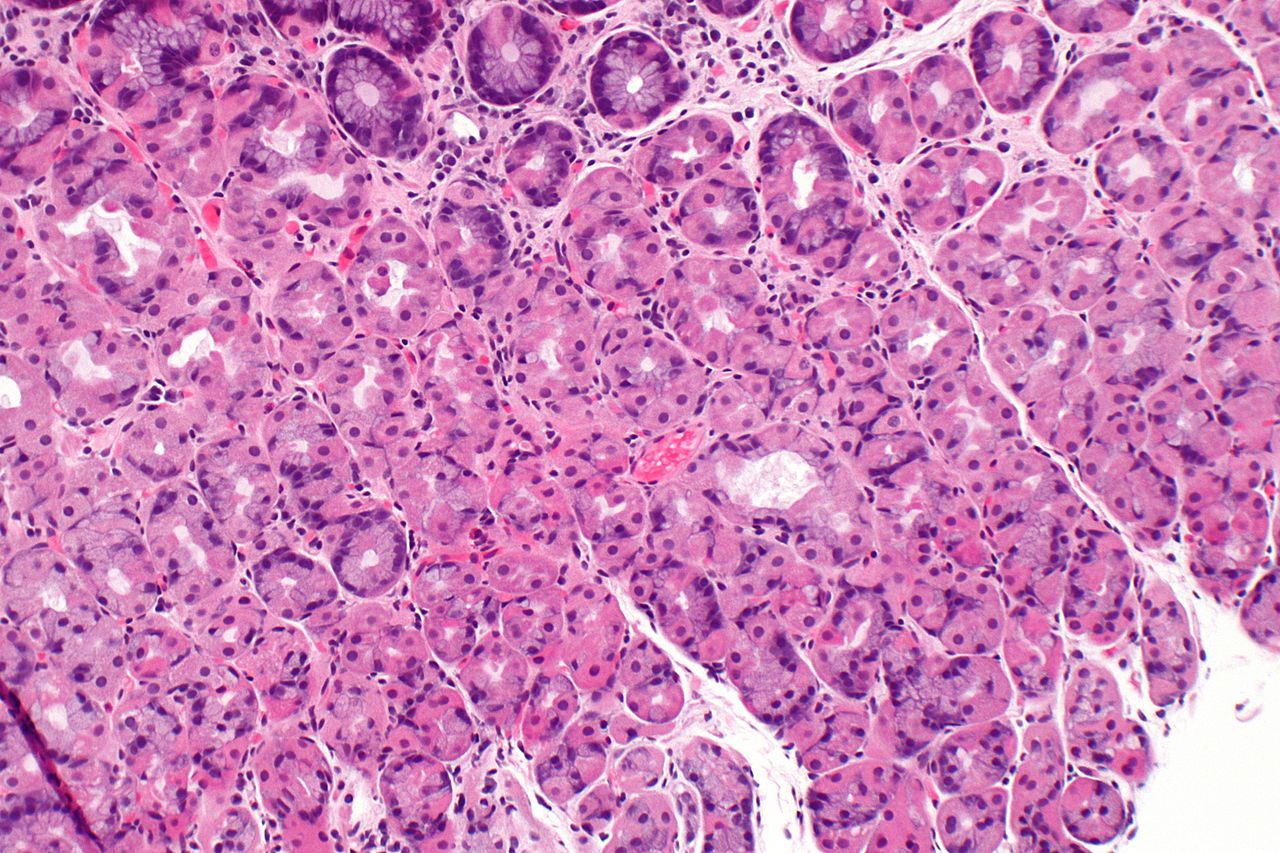
The humble honey bee has just 250 million base pairs in its genome. Aedes aegypti, the mosquito that carries yellow fever and dengue fever, has so many more—1.3 billion base pairs, about as many as the eastern spot-billed duck. Cows have more still, about 2.7 billion base pairs, while we have about 3.2 billion. And then there’s the migratory locust, which beats out every other species (at least among the ones whose genomes we’ve sequenced) with a whopping 5.8 billion base pairs. If you were to rank these animals on how complex they are, you wouldn’t base your list on the size of their genomes—a cow or a human is arguably more complex than a locust, and ducks are clearly more complex than mosquitos.
“If you look at an earthworm and compare it to a human, you look more complex, you have different organs, you have different cell types,” says Colin Sharpe, a genetics researcher at the University of Portsmouth, and the coauthor of a recent study that examines the connection between genes and complexity. The commonly accepted way to measure complexity, says Sharpe, is to count the number of different cells types in an animal—muscle cells, skin cells, neurons, and so on. Caenorhabditis elegans, a nematode worm frequently used in biology labs, has just 29 different cell types in its small body, while humans have about 170. What Sharpe and a graduate research partner, Daniela Lopes Cardoso, wanted to know is what it is about our genes that give us so many different kinds of cells.
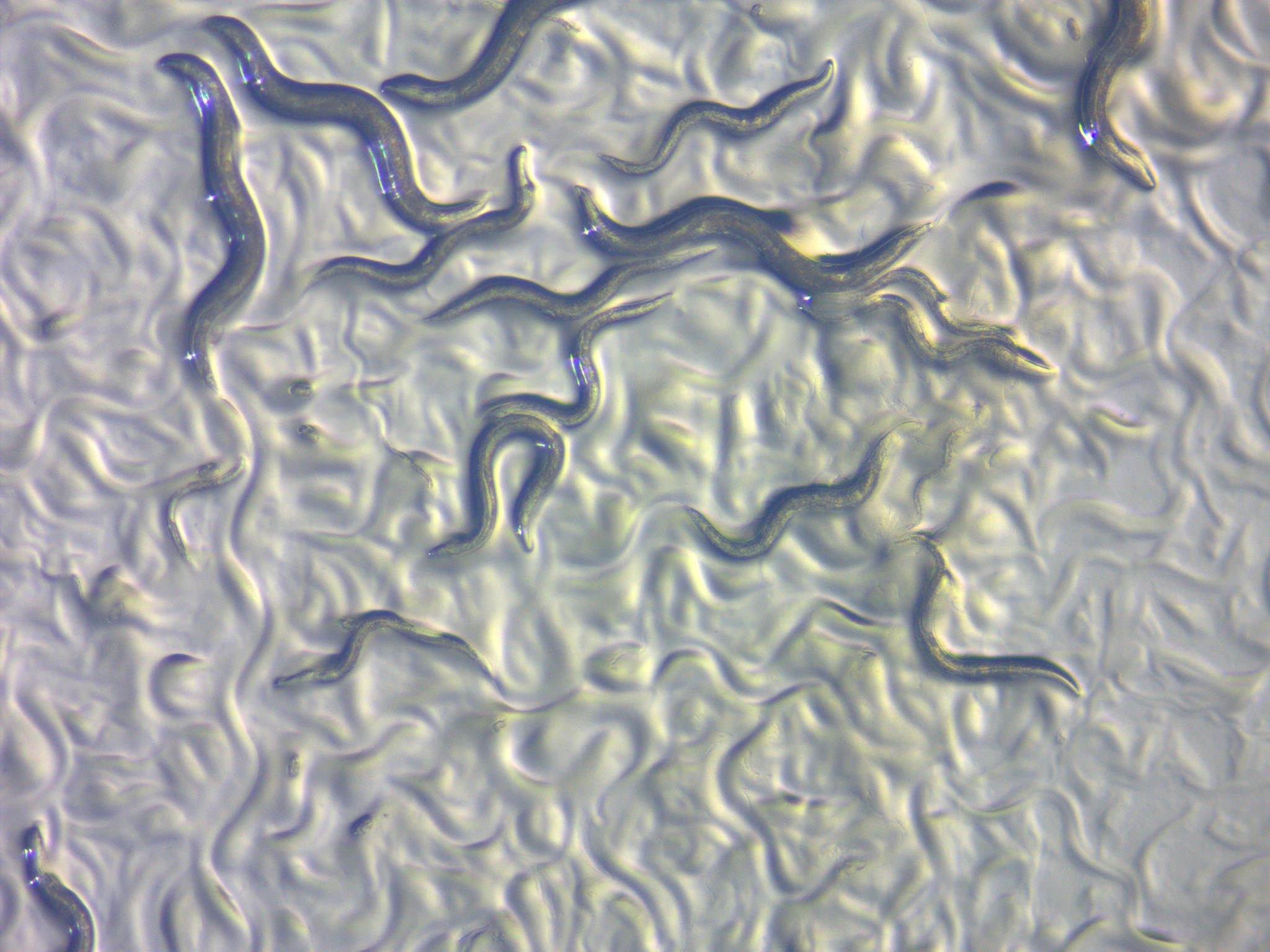
To do that, Cardoso and Sharpe used genome databases for organisms, including C. elegans and humans, to look for genes that are responsible for translating the genome into proteins. In more complex organisms, certain genes get repeated or translated a little differently, leading to more possible proteins encoded by a single gene, something called functional diversity. The scientists based their study on 2,000 human genes, and their equivalent genes in other organisms, to see how such functional diversity varies from species to species, and then looked at whether that change correlates with the number of cell types.
Certain genes tend to encode for more proteins in the more complex animals, and what those genes had in common was surprising. “What we thought we might find were the [genes for] proteins which directly regulate how genes are expressed,” says Sharpe. “Instead, it was the ones that regulate the structure and architecture of the chromatin and how it changes.” Chromatin is everything that forms the chromosomes in our cells—DNA and proteins—which means that the way our genetic material is packaged is what makes us more complex than worms.
Understanding what drives complexity may one day help biologists pick the optimal organism to use in their labs to model human diseases. “We were a bit concerned that if you take a very simple organism, and use it as a model system, it may not be a true reflection because it’s a much more simple organism,” says Sharpe. “If [a biological] process is much more complicated in humans, it wouldn’t be worth using a fruit fly as a model, you’d have to look at something more complicated, something like a mouse or a frog.” More research, he hopes, could lead to a system for picking the most appropriate model organism, with the right level of complexity. Thanks to better computing power, he plans to look at far more than 2,000 genes in the next iteration of the study. “Now we want to look at everything.”
Follow us on Twitter to get the latest on the world's hidden wonders.
Like us on Facebook to get the latest on the world's hidden wonders.
Follow us on Twitter Like us on Facebook