To Make a Field Guide to Life on Mars, First Head to the Deep Sea
Some scientists believe this is how we’ll know what to look for.
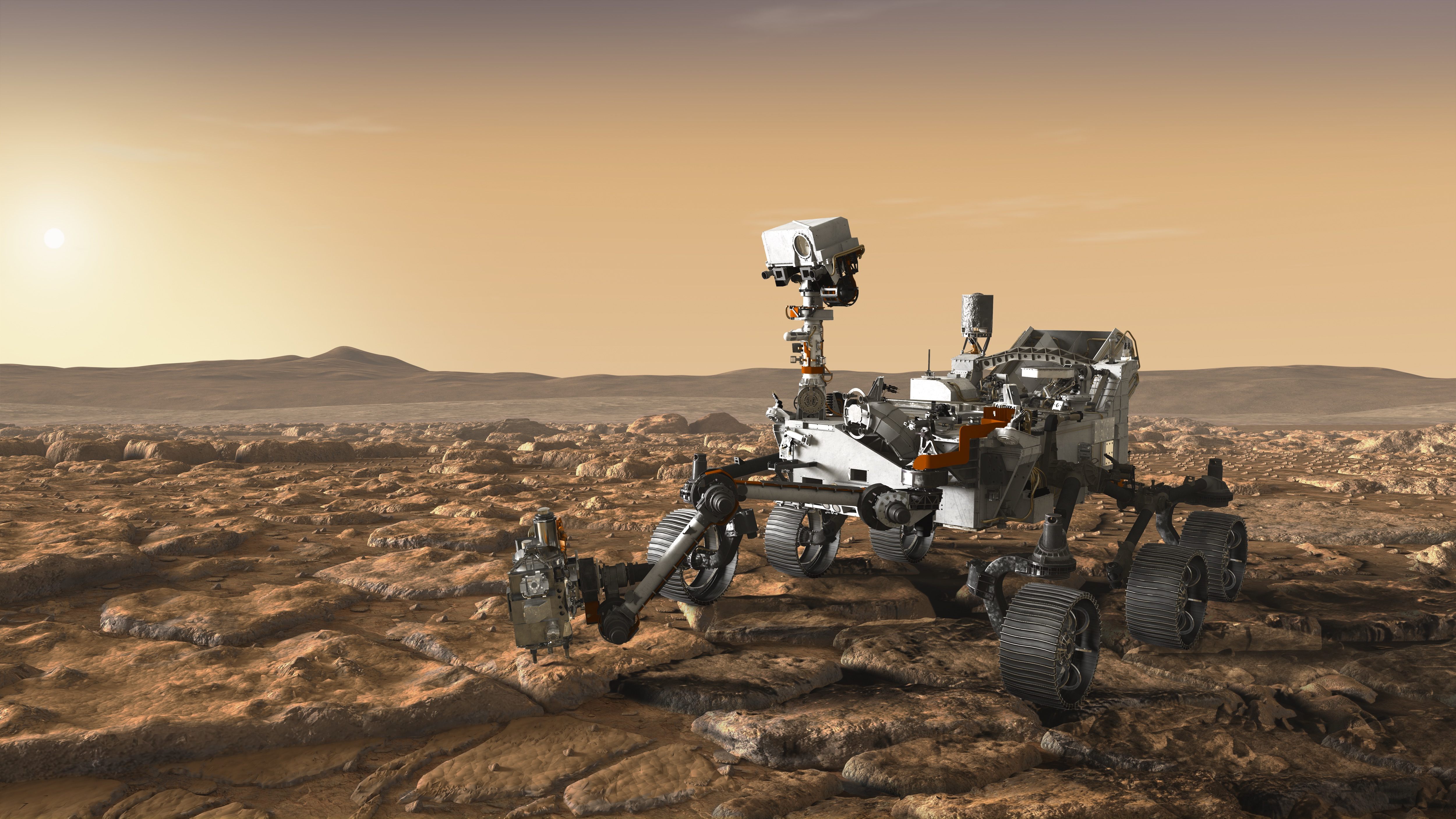
In 2021, a NASA rover will touch down on Mars in search of signs of life, past or present. It will investigate the surface of the red planet and collect samples from areas that seem particularly promising. But traces of life on Mars—if they exist—aren’t going to be apparent to the naked eye: Obviously there’s no remains of mammoths or goldfish or snails. Any record of life on Mars would likely take the form of organic compounds, which have already been identified up there but aren’t definitive, or actual fossils of microorganisms. Such fossils exist here on Earth, but they’re very tricky to spot—even in places we know they’ll be. The best strategy for finding these miniscule traces, according to a group of Scandinavian scientists, is to study the denizens of the deep sea. This team now plans to create an atlas of fossilized microbes from Earth’s oceans—an extraterrestrial field guide of sorts—to help the rover and its human partners identify definitive proof of life on Mars, according to their recent article in Frontiers in Earth Science.
From his lab in Stockholm, Magnus Ivarsson, a paleobiologist at the University of Southern Denmark and the Swedish Museum of Natural History, studies fossilized microbes locked in deep-sea volcanic rock. It’s a specialized area of study with little fanfare. “We’d been working on these type of fossils for 15 years but had never really thought of them as being interesting beyond Earth,” he says. But after attending a conference with researchers from NASA’s Jet Propulsion Laboratory, Ivarsson realized the space agency had a glaring lack of knowledge about fossils found in volcanic rock—the exact things they ought to be looking for off-world. “That’s when I started to realize how important our fossils are for the exploration of potential life on Mars,” he says.
On Earth, most fossils that scientists study are immortalized in sedimentary rock, which forms by the accumulation of small particles over time and encompasses 75 percent of the planet’s surface. Sedimentary rock is almost casual, with none of the heat and pressure that form metamorphic or igneous rocks, which is one thing that makes it particularly adept at preserving fossils. But Mars is home almost exclusively to volcanic rock. “Mars is a huge volcano, so to speak,” Ivarsson says. “It’s not as complex as Earth, from a geological point of view.”
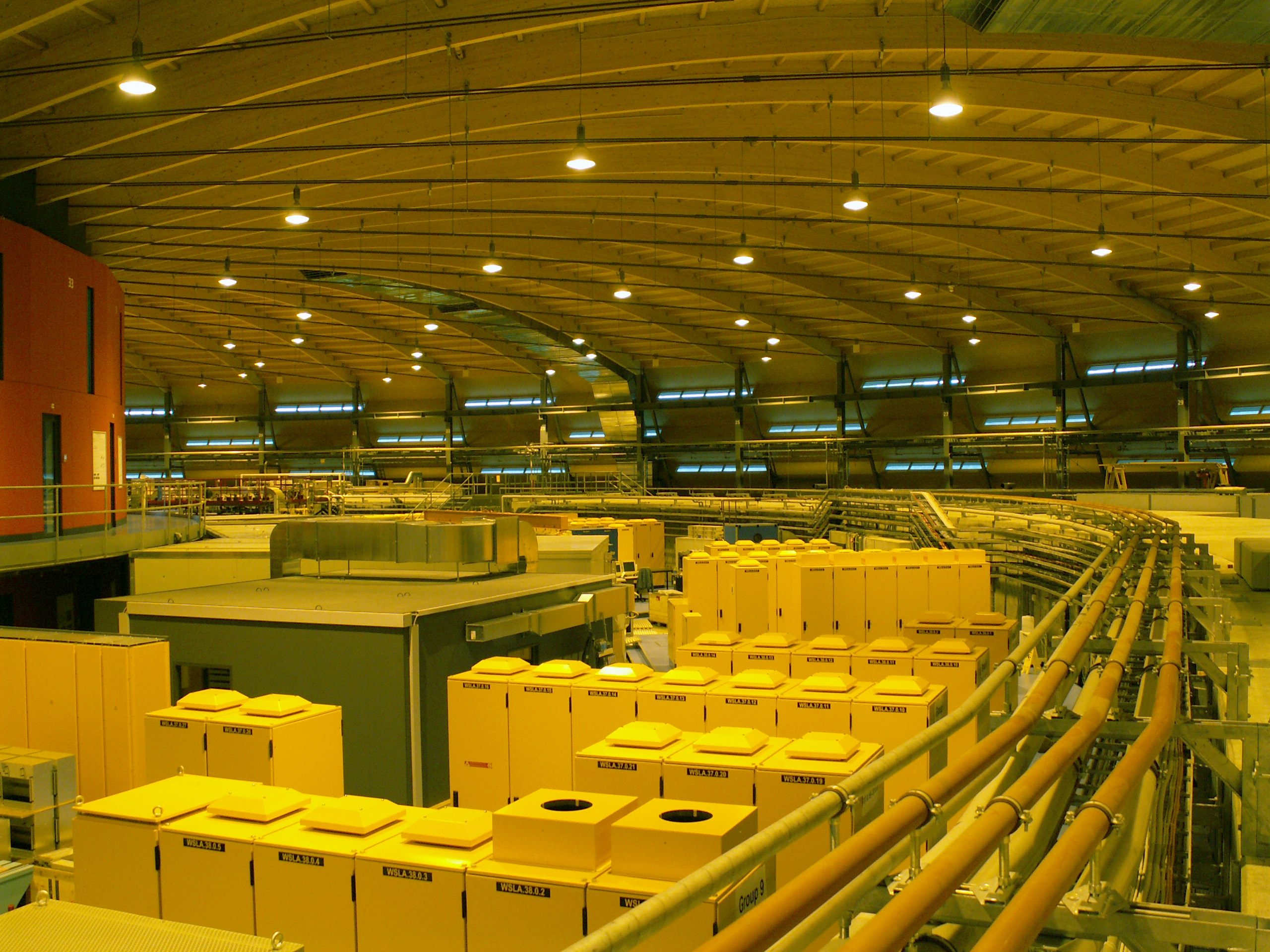
Despite having once been, you know, molten, volcanic rock can indeed contain fossils. But identifying them is not as simple as spotting the whorl of a shell, the veins of a leaf, or the familiar knobs of a prehistoric bone. These rocks are porous, riddled with tiny bubbles that create a network traversable by microbial life. When seawater and other fluids sweep through these channels, they bring microbes with them, which build colonies and self-sustaining food webs around the boundaries of the rock and in open pore space. After death, the microbes can be mineralized and fossilized in place. These are the creatures that Ivarsson studies, and the ones he believes may be most analogous to what scientists could find on Mars.
Ivarsson’s work identifying Earth’s microfossils, and the distinguishing traits that might help planetary scientists, is no easy feat. For one, they’re mostly found in the deep seafloor and must be collected by a specialized drill rig. These rigs can extend thousands of feet down and then pull a core back up to the surface—well, whatever portion doesn’t immediately fall back to the bottom. “We recover 30 to 40 percent, if we’re lucky,” Ivarsson says. His lab sources its cores from around the world, usually ordering them from repositories in Texas, Germany, and Japan. Most come from the Hawaiian–Emperor seamount chain, a vast underwater trail of volcanic islands and underwater mountains that extends from Hawaiʻi to the Russian coast.
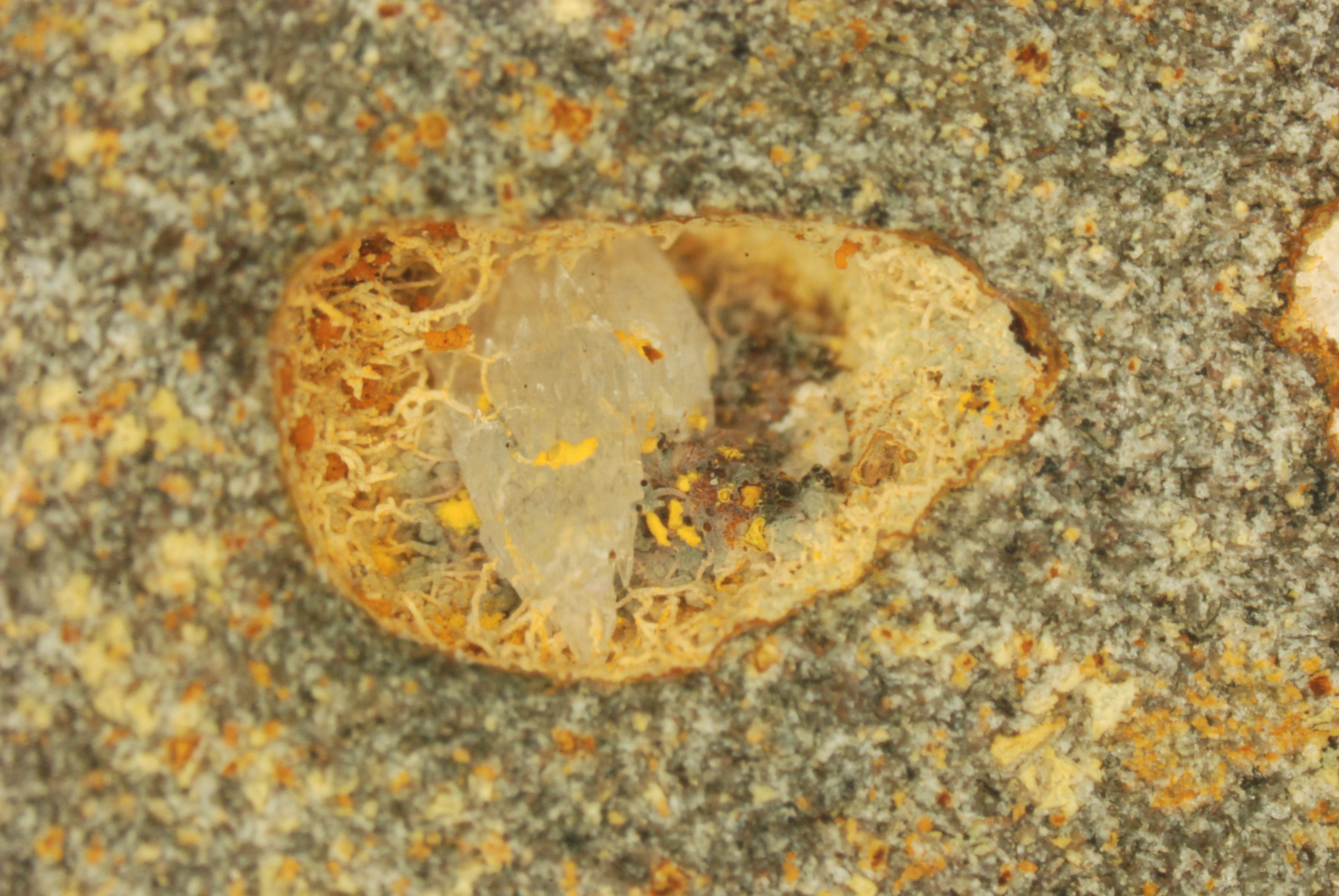
Once the cores arrive at the lab, the researchers store them in long boxes and study their stratigraphy, the accumulated layers, which can go back to around 80 or 90 million years ago. Then comes actually finding fossils. First, they examine the cores to identify interesting spots, such as fractures, veins, or, if they’re lucky, fossilized microbial communities large enough to be seen by the naked eye. Then they scan these promising spots with an electron microscope or X-ray for a closer look. It’s impossible to look over an entire core at that level of detail, just as one can’t examine an entire field of Martian rocks, so knowing what evidence of life can come from a targeted search is likely to be most helpful on the surface of Mars as well.
The microbes themselves are often just a couple of micrometers in length, a little smaller than a single strand of spider silk. But while a lone microbe is too tiny to be seen by the human eye, colonies of accumulated microbes can be much easier to spot. Take the bacteria Sulfurihydrogenibium yellowstonense, commonly known as Sulfuri, which grows up to three micrometers long. Colonies of Sulfuri can span an entire centimeter, Ivarsson says. Aside from their relatively large size, colonies of Sulfuri also have unusual, distinctive, noodle-like forms, as broad as fettucini or as fine as capellini. Sulfuri do not dwell in volcanic rocks like the microbes Ivarsson studies and plans to include in the atlas, but rather are found in hot springs such as those in Yellowstone National Park. But they also represent a good analogue for possible Martian life, since ancient volcanoes there could also have produced something like those geothermal features, according to a research team from the University of Illinois.
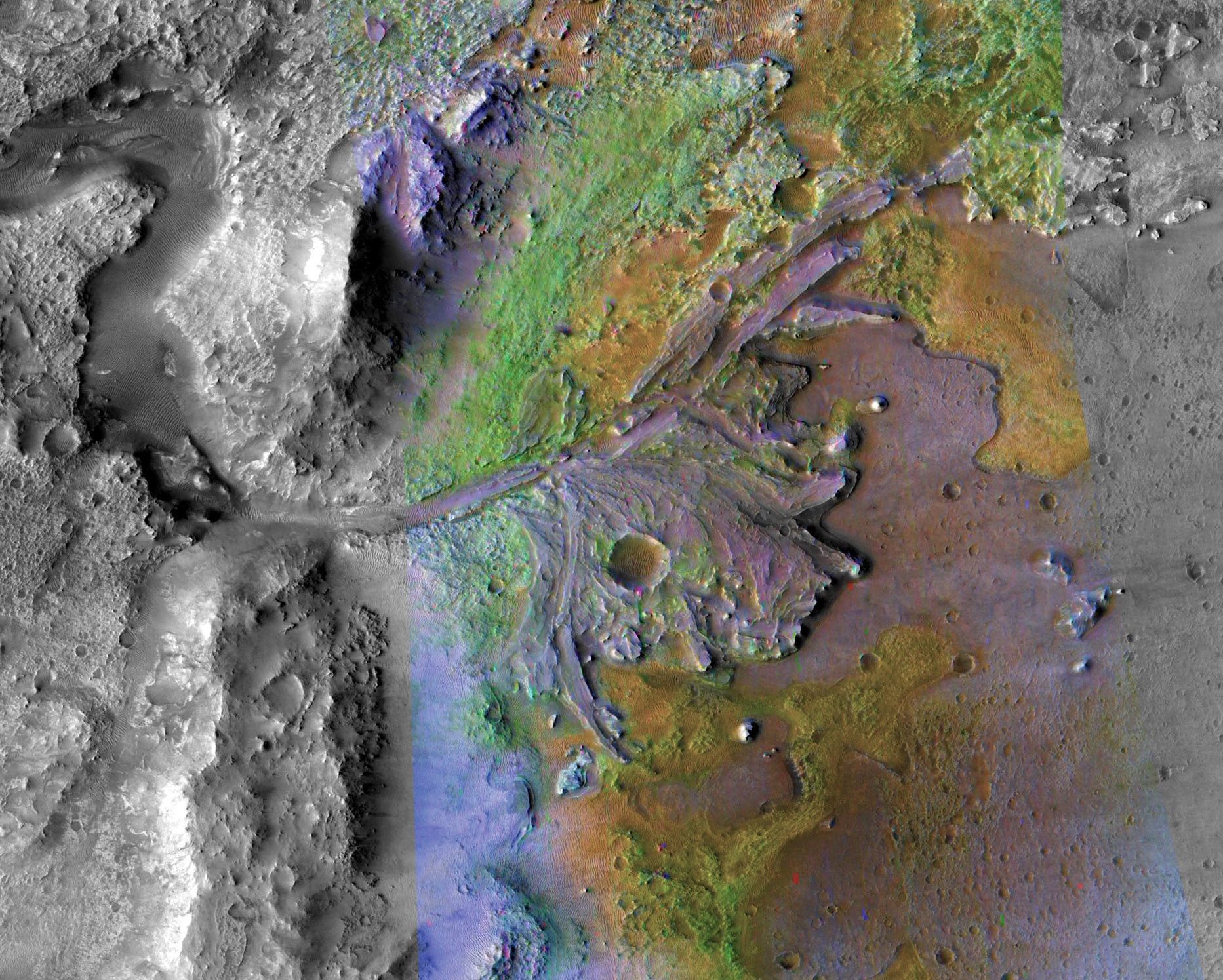
Right now the atlas is a plan, not a reality. “We’re trying to compile as much data as we can, going through the microfossils we see and making illustrations of them,” Ivarsson says, adding that he hopes the atlas will offer NASA researchers guidelines for how to identify promising spots and know what to look for in samples. He believes it will take the form of an enormous database, accessible to anyone, as well as a review in a scientific journal.
The timeline is somewhat tight, however, as NASA will launch its rover into space in July 2020, for a landing on the Jezero Crater on February 18, 2021. That will be a big year for the red planet. ExoMars, a rover from Europe and Russia, will also attempt the interplanetary trek. But Ivarsson believes his atlas will be useful for missions beyond, as well, such as when NASA attempts, someday, to bring Martian cores back to Earth for up-close examination. He doesn’t know which labs will have access to this material when it arrives, but he hopes to get at least a peek.
Follow us on Twitter to get the latest on the world's hidden wonders.
Like us on Facebook to get the latest on the world's hidden wonders.
Follow us on Twitter Like us on Facebook