DIY Tech Is Helping Scientists Keep Up With Marine Life
Marine biologists are engineering creative new ways to ride along with whales, bob with jellies, and drift with plankton.

It’s hard to conduct research in the ocean. Electronics don’t mix well with salt water and waves knock things around. The deeper you go, the darker and colder it gets. Pressure mounts to crushing levels. Oceans account for 70 percent of Earth’s surface and more than 99 percent of its biosphere, but only about 10 or 15 percent of it has been mapped with any rigor, estimated curator and ichthyologist John Sparks at a recent tour of the American Museum of Natural History’s new Unseen Oceans exhibition. “We know more about the surface of Mars than the ocean floor,” he said.
When scientists want to track how the tiniest microscopic plants or the largest of whales move through, they often find themselves without the right tools for the job. Whether looking at creatures that are carried by currents, propel themselves slowly through bays, or cross long distances of open ocean, researchers often have to make their own machines and devise ad-hoc solutions, added Michael Novacek, the museum’s provost of science and the curator of the division of paleontology. “It’s not stuff that’s off the shelf.”
Researchers have to rig up rugged, inexpensive, custom tools and sensors that are both flexible and adaptable. (Take, for instance, a robotic fish that can mix in with schools or squishy-fingered grippers that can grab corals with minimal damage.) Sparks refers to this breed of scientists as tinkerers. “They build their own equipment, they solve their own problems,” he said. Here’s how some of them have engineered ways to keep track of creatures on the move.
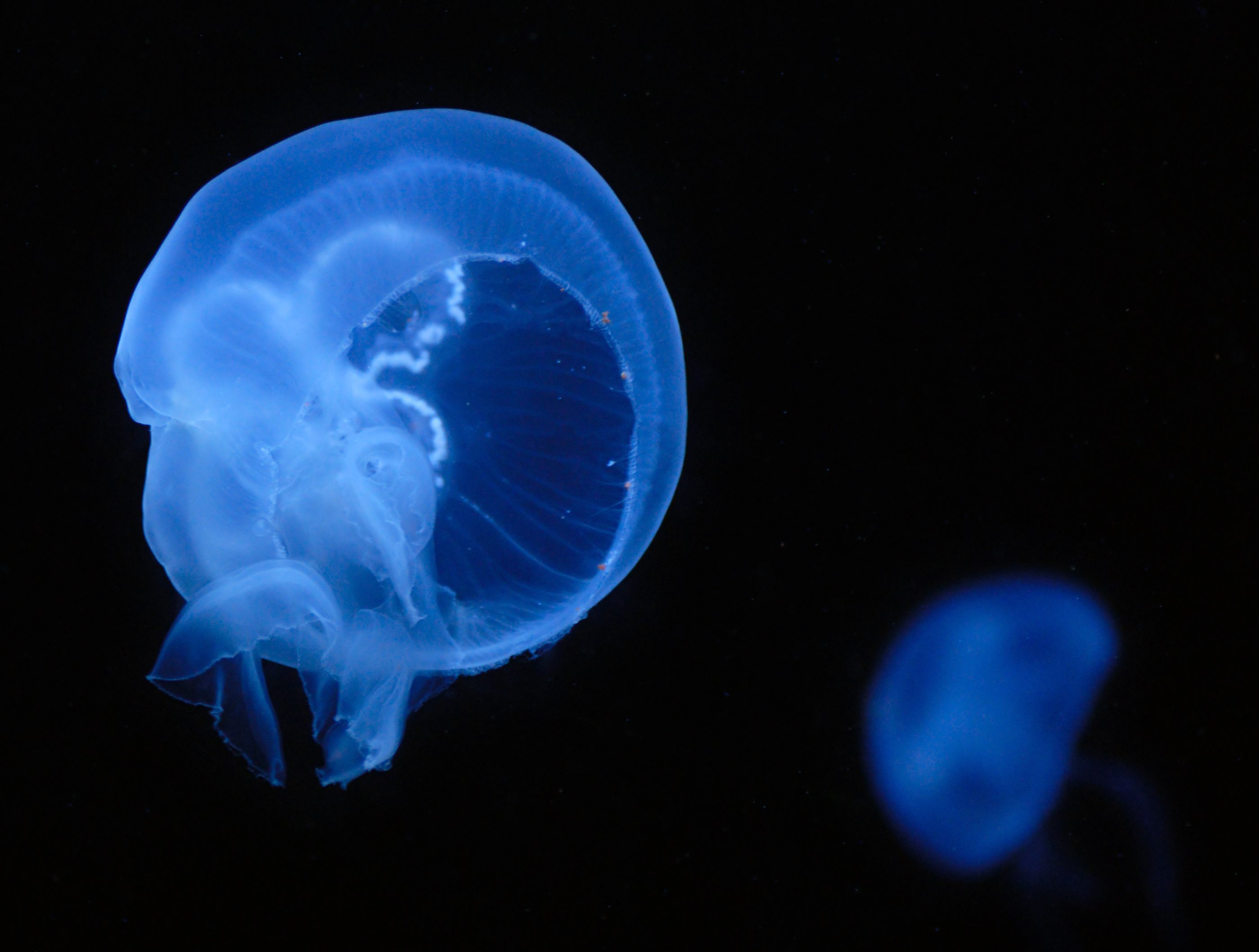
How to hang with jellyfish
Kakani Katija, a principal engineer at the Monterey Bay Aquarium Research Institute, wants to know what moon jellies are up to. How do they behave in their natural environment? Do they linger in areas where food is plentiful, or are they completely subject to currents? To answer questions like these, Katija needs data—acceleration, pressure, depth, and temperature.
The trouble is that the qualities that make jellies look so ethereal also make them hard to handle or track. They are delicate and neutrally buoyant, so researchers are reluctant to saddle them with devices that can drag them down. Off-the-shelf tags and sensors tend to be too bulky and heavy for jellies. Scientists also want to make sure that their interventions aren’t sabotaging their data. “If you’re interested in behavior, how do you ensure that the way you’re attaching [a device] doesn’t change behavior?” Katija asks. To control for this, a data-collecting tag needs to be small, unobtrusive, and aerodynamic. So Katija and her colleagues collaborated with Florida-based Loggerhead Instruments to engineer a slim tag using open-source models. The next hurdle was finding a way to affix it to a diaphanous creature.
Some researchers had tried affixing equipment to jellies with zip ties, but not all of them have the right kind of protuberances to attach them to. Katija and her collaborators started playing around with different materials and adhesives, and collaborated with various aquariums to try them out on animals in tanks. One of the best solutions for attaching the tag, it turned out, was in Katija’s backpack the whole time.
Katija keeps a stash of vet glue, for treating cuts, on hand in case her dog gets injured while they’re out hiking. That same glue has proved to be a promising way to adhere sensors to the jellies. The glue holds tight, and then sloughs off in a few hours or days—the timeline is still up in the air. They sensors are strung on monofilament line and tied to a float, so the researchers can use GPS to collect them.
The tags will hit the water of Monterey Bay next month, just in time for a jellyfish bloom.
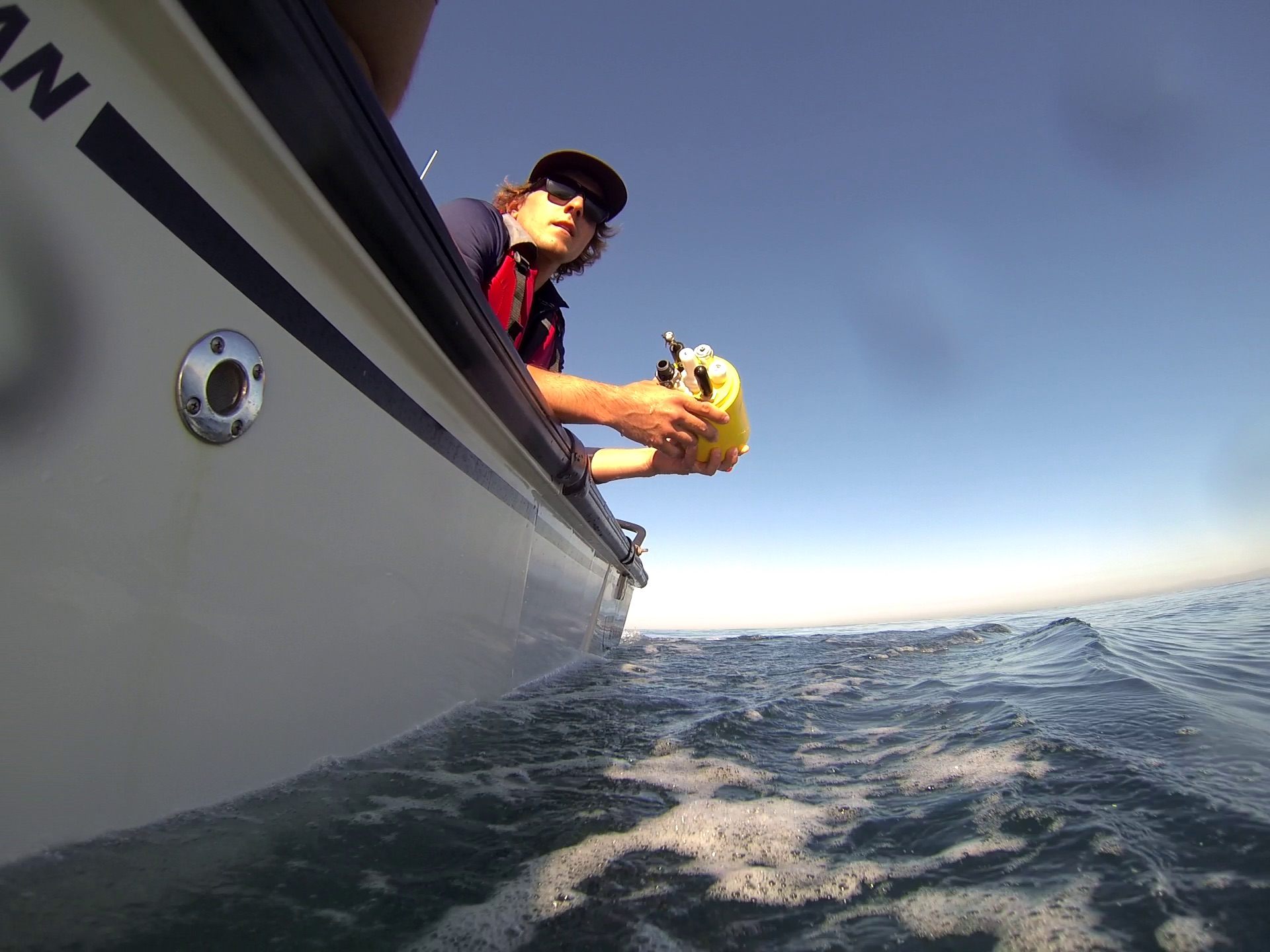
DIY drifting with plankton
Many sea creatures—fish, whales, and the like—can swim against a current. Not plankton. These microscopic plants and animals, which provide the base of the marine food chain and are major sources of atmospheric oxygen, are dedicated drifters who go where the currents take them. Despite their tiny size, their fate matters a great deal. “If the phytoplankton crash, there goes our oxygen,” said Sparks.
Jules Jaffe, a research oceanographer at the Marine Physical Laboratory at Scripps Institution of Oceanography, University of California, San Diego, wanted to get a better sense of how and where plankton move. His “autonomous miniature underwater robot drifters” are proxies for the tiny organisms themselves. Composed of concentric foam cylinders around suite of sensors collecting temperature, pressure, and more, these floaters are tossed over the side of a small boat. Then they ride underwater currents, just like plankton.
Researchers use sound to track the devices, which stay in the water for a few hours or a couple of days at a time. So far Jaffe has used them off of La Jolla, California, and the Cayman Islands, where they were used to study grouper embryos.
“These guys aren’t bad, but they’re not exactly like a plankton,” Jaffe says. For one thing, they are considerably larger, which effects the drag on them as they travel through the water. “If the water is moving slowly enough, presumably their drag coefficient is high enough that we can get meaningful data from them,” he says. “If the water was really accelerating, they might be left behind a little bit, and then we would worry about that.”
Jaffe looks forward to more tinkering—to a 3-D-printed future brimming with “smaller, cheaper, faster things.” But he’s already pretty amazed by how his devices have provided a never-before-seen view into the planktonic world. “Nobody would have seen this before unless you put yourself in their reference frame,” he says. “We are coexisting with these different scales of life, and the physics surrounding them actually changes the way they experience the world.”
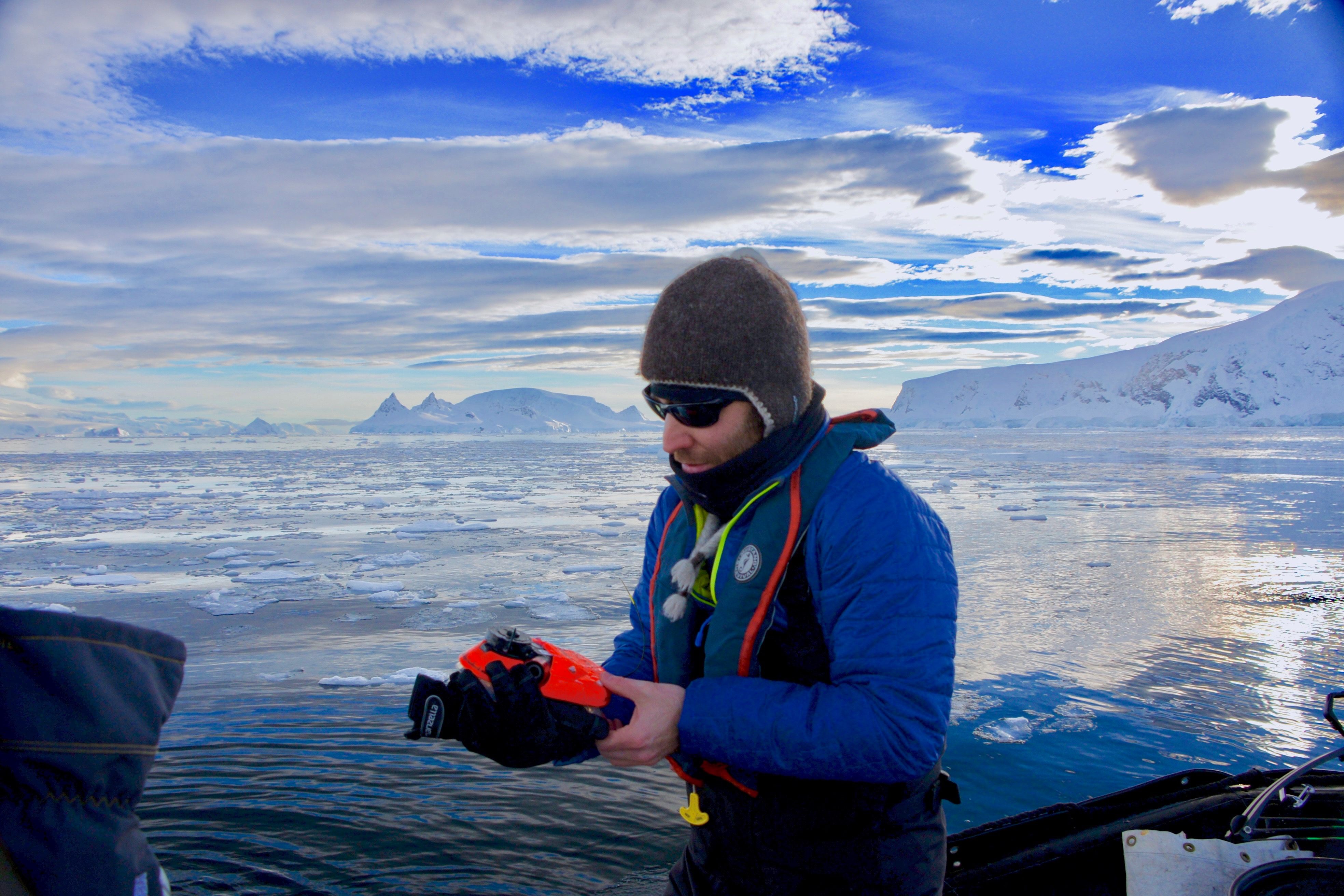
Tagging along with whales
A researcher stands at the front of a boat, grappling with a long pole that dangles into the water. There’s a whale up ahead, a flash of a fin or a plume of spray. The researcher stretches the pole out just a little farther, and makes contact. With suction cups, he adheres a collection of lightweight sensors to the whale’s back. When the cetacean dives back underwater, a whole scientific crew is going along for the ride.
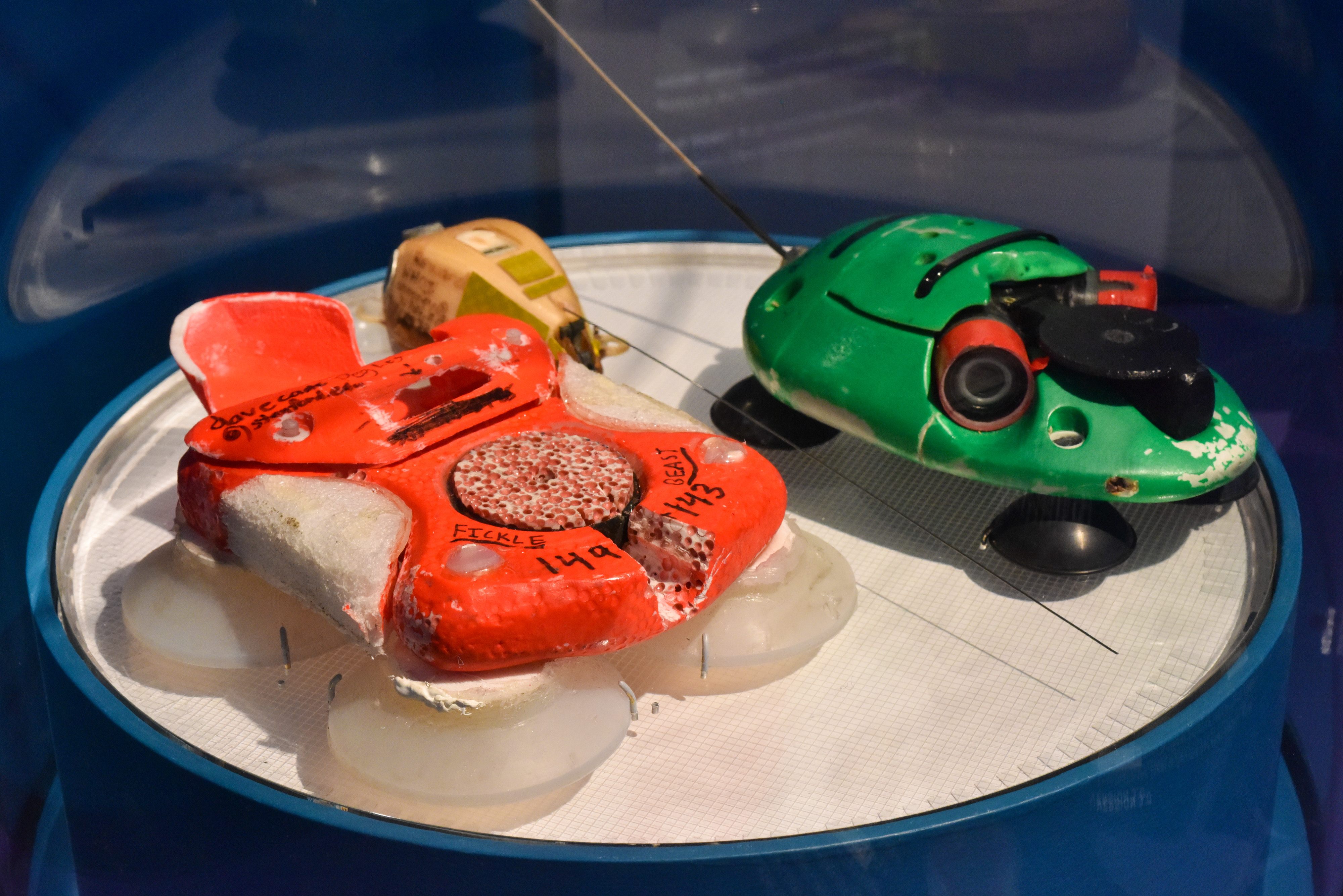
With his collaborators, Jeremy Goldbogen, an assistant professor of biology at Stanford University’s Hopkins Marine Station, devised tags that contain both miniaturized movement sensors—accelerometers, magnetometers, and more—and video recorders. The team managed to shrink these to fit a piece of styrofoam a little larger than a human hand. The sensors gather data, and the video feed lets the researchers ride shotgun.
The suction cups proved to be an ingenious way to adhere the whole thing to a whale’s smooth body, and critical for studying species that surface briefly and spend long stretches underwater. You might only have a few chances to make the sensors stick. The team is currently tagging minke whales off the Antarctic Peninsula, where they’re learning more about movement patterns, eating habits, and day-to-day life in the ocean’s blue-green depths.
Follow us on Twitter to get the latest on the world's hidden wonders.
Like us on Facebook to get the latest on the world's hidden wonders.
Follow us on Twitter Like us on Facebook